Interaction of biological, chemical and environmental factors in Biodegradation processes
Introduction to Biodegradation
Biodegradation is the process by which organic substances are broken down by living organisms, primarily microorganisms, into simpler compounds. This process is essential for the recycling of nutrients in ecosystems and the removal of pollutants from the environment. The efficiency and outcome of biodegradation depend on a complex interplay of biological, chemical, and environmental factors.

Biological Factors
Microbial Communities:
- Diversity and Composition: Different microbial species possess varying abilities to degrade specific compounds. A diverse microbial community enhances the potential for biodegradation due to the presence of multiple enzymatic pathways.
- Adaptation and Acclimation: Microorganisms can adapt to the presence of pollutants, developing or upregulating specific enzymes required for degradation.
- Population Density: Higher concentrations of microorganisms can enhance the rate of biodegradation due to increased enzymatic activity.
Enzymatic Activity:
- Degradative Enzymes: Microorganisms produce specific enzymes that catalyze the breakdown of complex organic molecules. These enzymes include oxidases, dehydrogenases, and hydrolases.
- Induction and Regulation: The production of degradative enzymes can be induced by the presence of specific substrates (pollutants), regulated by genetic and environmental factors.
Chemical Factors
Substrate Concentration:
- Bioavailability: The availability of the pollutant to the microorganisms is crucial. Pollutants that are dissolved or easily accessible are degraded more readily than those that are adsorbed to soil particles or trapped within complex matrices.
- Toxicity: High concentrations of certain pollutants can be toxic to microorganisms, inhibiting biodegradation processes.
Chemical Structure:
- Complexity and Reccalcitrance: Simple organic molecules are generally degraded more easily than complex or recalcitrant compounds such as polycyclic aromatic hydrocarbons (PAHs) or chlorinated solvents.
- Functional Groups: The presence of functional groups like halogens, nitro groups, or methyl groups can affect the ease of degradation. For example, chlorinated compounds are often more resistant to biodegradation.
Nutrient Availability:
- Carbon Source: Pollutants often serve as a carbon source for microorganisms. The presence of additional, more readily degradable carbon sources can either enhance or inhibit the biodegradation of specific pollutants.
- Nitrogen and Phosphorus: These nutrients are essential for microbial growth and metabolism. A balanced ratio of carbon, nitrogen, and phosphorus (often C:N= 100:10:1) is typically required for optimal biodegradation.
Environmental Factors
Temperature:
- Optimal Range: Each microorganism has an optimal temperature range for growth and enzymatic activity. Generally, biodegradation rates increase with temperature up to an organism's optimal range, beyond which enzymes may denature, and microbial activity decreases.
- Seasonal Variations: In natural environments, seasonal temperature fluctuations can significantly impact biodegradation rates.
pH:
- Optimal pH: Most biodegradation processes are optimal within a neutral pH range (6-8). Extreme pH levels can inhibit microbial growth and enzyme activity.
- Buffering Capacity: The presence of buffering agents in the environment can help maintain a stable pH conducive to biodegradation.
Oxygen Availability:
- Aerobic vs. Anaerobic Conditions: Biodegradation can occur under both aerobic (oxygen-present) and anaerobic (oxygen-absent) conditions, but the pathways and rates differ. Aerobic biodegradation is generally faster and more complete but requires sufficient oxygen supply.
- Redox Potential: The redox potential of the environment influences the types of microorganisms present and the degradation pathways they utilize.
Moisture Content:
- Water Availability: Adequate moisture is necessary for microbial metabolism and transport of nutrients and pollutants. Too little moisture can limit microbial activity, while excessive moisture can create anaerobic conditions.
- Hydraulic Conductivity: In soil and sediment environments, the movement of water affects the transport of pollutants and nutrients, influencing biodegradation rates.
Interactions Among Factors
The interaction between biological, chemical, and environmental factors is complex and dynamic. For example, the bioavailability of a pollutant can be influenced by pH, which in turn affects microbial activity. Similarly, the presence of co-contaminants can alter the microbial community structure and enzymatic pathways involved in biodegradation.
Case Study: Biodegradation of Petroleum Hydrocarbons
- Biological Factors: Native microbial populations in contaminated soils or water bodies can degrade petroleum hydrocarbons. Specific bacteria (e.g., Pseudomonas, Acinetobacter) and fungi (e.g., Aspergillus, Penicillium) are known for their hydrocarbon-degrading capabilities.
- Chemical Factors: Hydrocarbon composition (e.g., alkanes, aromatics), concentration, and the presence of other nutrients (e.g., nitrogen, phosphorus) affect biodegradation rates. Some hydrocarbons are more readily degraded than others.
- Environmental Factors: Optimal temperature (25-30°C), neutral pH, aerobic conditions, and adequate moisture content are essential for efficient hydrocarbon degradation. Oil spills in marine environments often require biostimulation (addition of nutrients) or bioaugmentation (addition of specific microbial strains) to enhance biodegradation.
Interaction of biological, chemical and environmental factors in Biodegradation processes
Introduction to Biodegradation
Biodegradation is the process by which organic substances are broken down by living organisms, primarily microorganisms, into simpler compounds. This process is essential for the recycling of nutrients in ecosystems and the removal of pollutants from the environment. The efficiency and outcome of biodegradation depend on a complex interplay of biological, chemical, and environmental factors.

Biological Factors
Microbial Communities:
- Diversity and Composition: Different microbial species possess varying abilities to degrade specific compounds. A diverse microbial community enhances the potential for biodegradation due to the presence of multiple enzymatic pathways.
- Adaptation and Acclimation: Microorganisms can adapt to the presence of pollutants, developing or upregulating specific enzymes required for degradation.
- Population Density: Higher concentrations of microorganisms can enhance the rate of biodegradation due to increased enzymatic activity.
Enzymatic Activity:
- Degradative Enzymes: Microorganisms produce specific enzymes that catalyze the breakdown of complex organic molecules. These enzymes include oxidases, dehydrogenases, and hydrolases.
- Induction and Regulation: The production of degradative enzymes can be induced by the presence of specific substrates (pollutants), regulated by genetic and environmental factors.
Chemical Factors
Substrate Concentration:
- Bioavailability: The availability of the pollutant to the microorganisms is crucial. Pollutants that are dissolved or easily accessible are degraded more readily than those that are adsorbed to soil particles or trapped within complex matrices.
- Toxicity: High concentrations of certain pollutants can be toxic to microorganisms, inhibiting biodegradation processes.
Chemical Structure:
- Complexity and Reccalcitrance: Simple organic molecules are generally degraded more easily than complex or recalcitrant compounds such as polycyclic aromatic hydrocarbons (PAHs) or chlorinated solvents.
- Functional Groups: The presence of functional groups like halogens, nitro groups, or methyl groups can affect the ease of degradation. For example, chlorinated compounds are often more resistant to biodegradation.
Nutrient Availability:
- Carbon Source: Pollutants often serve as a carbon source for microorganisms. The presence of additional, more readily degradable carbon sources can either enhance or inhibit the biodegradation of specific pollutants.
- Nitrogen and Phosphorus: These nutrients are essential for microbial growth and metabolism. A balanced ratio of carbon, nitrogen, and phosphorus (often C:N= 100:10:1) is typically required for optimal biodegradation.
Environmental Factors
Temperature:
- Optimal Range: Each microorganism has an optimal temperature range for growth and enzymatic activity. Generally, biodegradation rates increase with temperature up to an organism's optimal range, beyond which enzymes may denature, and microbial activity decreases.
- Seasonal Variations: In natural environments, seasonal temperature fluctuations can significantly impact biodegradation rates.
pH:
- Optimal pH: Most biodegradation processes are optimal within a neutral pH range (6-8). Extreme pH levels can inhibit microbial growth and enzyme activity.
- Buffering Capacity: The presence of buffering agents in the environment can help maintain a stable pH conducive to biodegradation.
Oxygen Availability:
- Aerobic vs. Anaerobic Conditions: Biodegradation can occur under both aerobic (oxygen-present) and anaerobic (oxygen-absent) conditions, but the pathways and rates differ. Aerobic biodegradation is generally faster and more complete but requires sufficient oxygen supply.
- Redox Potential: The redox potential of the environment influences the types of microorganisms present and the degradation pathways they utilize.
Moisture Content:
- Water Availability: Adequate moisture is necessary for microbial metabolism and transport of nutrients and pollutants. Too little moisture can limit microbial activity, while excessive moisture can create anaerobic conditions.
- Hydraulic Conductivity: In soil and sediment environments, the movement of water affects the transport of pollutants and nutrients, influencing biodegradation rates.
Interactions Among Factors
The interaction between biological, chemical, and environmental factors is complex and dynamic. For example, the bioavailability of a pollutant can be influenced by pH, which in turn affects microbial activity. Similarly, the presence of co-contaminants can alter the microbial community structure and enzymatic pathways involved in biodegradation.
Case Study: Biodegradation of Petroleum Hydrocarbons
- Biological Factors: Native microbial populations in contaminated soils or water bodies can degrade petroleum hydrocarbons. Specific bacteria (e.g., Pseudomonas, Acinetobacter) and fungi (e.g., Aspergillus, Penicillium) are known for their hydrocarbon-degrading capabilities.
- Chemical Factors: Hydrocarbon composition (e.g., alkanes, aromatics), concentration, and the presence of other nutrients (e.g., nitrogen, phosphorus) affect biodegradation rates. Some hydrocarbons are more readily degraded than others.
- Environmental Factors: Optimal temperature (25-30°C), neutral pH, aerobic conditions, and adequate moisture content are essential for efficient hydrocarbon degradation. Oil spills in marine environments often require biostimulation (addition of nutrients) or bioaugmentation (addition of specific microbial strains) to enhance biodegradation.
Bioremediation processes

Introduction to Bioremediation
Bioremediation is the process of using living organisms, primarily microorganisms, plants, and their enzymes, to degrade, detoxify, or transform hazardous contaminants in soils, sediments, water, and air into less harmful products. It is an environmentally friendly, cost-effective method for cleaning up polluted environments.

Types of Bioremediation
Bioremediation processes can be broadly classified into in situ (on-site) and ex situ (off-site) methods:
In Situ Bioremediation:
- Bioventing: This involves supplying air or oxygen to existing soil microorganisms. Nutrients may also be added to enhance microbial activity, promoting the aerobic degradation of contaminants.
- Biosparging: Similar to bioventing but used for groundwater contamination, biosparging involves injecting air or oxygen below the water table to increase groundwater oxygen concentrations and enhance the activity of naturally occurring bacteria.
- Bioaugmentation: Adding specific strains of microorganisms that can degrade contaminants to the polluted site to boost the degradation process.
- Phytoremediation: Using plants to absorb, concentrate, and/or degrade contaminants. Different types of phytoremediation include phytoextraction (uptake of contaminants by plant roots), phytostabilization (stabilization of contaminants in soil), and phytodegradation (degradation of contaminants by plant enzymes).
Ex Situ Bioremediation:
- Biopiles: Contaminated soils are excavated and placed into piles for treatment. The piles are aerated and sometimes supplemented with nutrients and moisture to enhance microbial activity.
- Landfarming: Contaminated soil is spread over a large area and periodically tilled to aerate the soil, enhancing microbial degradation of pollutants.
- Composting: Mixing contaminated soil with organic amendments (e.g., manure, agricultural wastes) to support microbial activity and degradation.
- Bioreactors: Contaminated soil or water is placed in a controlled reactor where conditions such as temperature, pH, oxygen, and nutrient levels can be optimized for microbial degradation.
Mechanisms of Bioremediation
Microbial Degradation:
- Aerobic Degradation: Microorganisms use oxygen to degrade organic pollutants, converting them into carbon dioxide, water, and biomass. This process is effective for petroleum hydrocarbons, phenols, and other organic contaminants.
- Anaerobic Degradation: In the absence of oxygen, microorganisms use alternative electron acceptors (e.g., nitrate, sulfate) to degrade contaminants. This process is suitable for chlorinated solvents and other recalcitrant compounds.
- Co-metabolism: Microorganisms degrade contaminants incidentally while metabolizing another compound. This process often requires the presence of a primary substrate to induce the necessary enzymes.
Phytoremediation Mechanisms:
- Phytoextraction: Plants absorb contaminants through their roots and accumulate them in above-ground tissues, which can be harvested and disposed of safely.
- Phytostabilization: Plants immobilize contaminants in the soil, preventing their migration and reducing bioavailability.
- Phytodegradation: Plants produce enzymes that can break down contaminants directly in the soil or within plant tissues.
- Rhizodegradation: Root exudates stimulate microbial activity in the rhizosphere (root zone), enhancing the biodegradation of contaminants.
Factors Affecting Bioremediation
Environmental Factors:
- Temperature: Optimal microbial activity typically occurs within a specific temperature range, often between 20-40°C.
- pH: Most biodegradation processes are optimal at a neutral pH (6-8). Extreme pH levels can inhibit microbial activity.
- Moisture Content: Adequate moisture is essential for microbial metabolism and nutrient transport. Too much or too little moisture can limit biodegradation efficiency.
- Oxygen Availability: Oxygen is crucial for aerobic degradation processes. Insufficient oxygen levels can slow down or inhibit degradation.
Nutrient Availability:
- Carbon, Nitrogen, and Phosphorus: A balanced ratio of these nutrients (often C:N= 100:10:1) is required for optimal microbial growth and activity.
- Trace Elements: Essential micronutrients such as magnesium, calcium, and iron are also necessary for microbial metabolism.
Contaminant Characteristics:
- Chemical Structure: Simple organic molecules are generally more easily degraded than complex or recalcitrant compounds (e.g., PAHs, PCBs).
- Concentration: High concentrations of certain contaminants can be toxic to microorganisms, inhibiting biodegradation.
- Bioavailability: Contaminants that are dissolved or easily accessible are more readily degraded than those that are adsorbed to soil particles or trapped within complex matrices.
Advantages of Bioremediation
- Environmentally Friendly: Uses natural processes to clean up pollutants without introducing harmful chemicals.
- Cost-Effective: Often less expensive than conventional methods such as incineration or chemical treatment.
- Versatile: Can be applied to a wide range of contaminants and environmental settings.
Limitations of Bioremediation
- Time-Consuming: Bioremediation can take longer than other remediation methods, especially for recalcitrant compounds.
- Site-Specific: The effectiveness of bioremediation depends on site-specific conditions such as soil type, climate, and contaminant characteristics.
- Incomplete Degradation: Some contaminants may not be fully degraded, leading to the formation of intermediate compounds that could be toxic.
Case Studies
Oil Spill Bioremediation:
- The Exxon Valdez oil spill in 1989 led to the development of bioremediation techniques for marine environments. Nutrient amendments were used to enhance the degradation of oil by indigenous microorganisms, significantly reducing the impact of the spill.
Phytoremediation of Heavy Metals:
- Phytoremediation has been successfully used to clean up heavy metal-contaminated sites. For example, Indian mustard (Brassica juncea) has been shown to accumulate lead, cadmium, and chromium from contaminated soils, allowing for the safe removal of these metals.
Bioreactors for Industrial Wastewater:
- Bioreactors have been employed to treat industrial wastewater containing organic pollutants. Controlled conditions in the bioreactor, such as pH, temperature, and nutrient levels, ensure efficient microbial degradation of contaminants.
Definition and classification including in situ and ex situ types
Definition of Bioremediation
Bioremediation is a process that uses living organisms, primarily microorganisms, fungi, plants, or their enzymes, to detoxify, degrade, and transform hazardous substances in the environment into less harmful or non-toxic forms. This technology leverages natural biological processes to remediate contaminated soil, water, and air, offering an environmentally friendly and often cost-effective alternative to traditional physical and chemical remediation methods.
Classification of Bioremediation
Bioremediation techniques can be broadly classified into two main categories based on where the remediation process takes place: in situ and ex situ. Each category encompasses various methods tailored to specific types of contaminants and environmental conditions.

1. In Situ Biodegradation
In situ biodegradation occurs directly at the site of contamination without removing the contaminated material. This method is often preferred for treating large areas of contaminated soil or groundwater because it is less disruptive and more cost-effective than ex situ methods.
Types of In Situ Biodegradation:

- Natural Attenuation: This process relies on natural environmental conditions and indigenous microorganisms to degrade contaminants without human intervention.
- Bioventing: Bioventing techniques involve controlled stimulation of airflow by delivering oxygen to unsaturated (vadose) zone in order to increase activities of indigenous microbes for bioremediation. In bioventing, amendments are made by adding nutrients and moisture to increase bioremediation. That will achieve microbial transformation of pollutants to a harmless state.
-

- Bioslurping: This technique combines vacuum-enhanced pumping, soil vapor extraction and bioventing to achieve soil and ground water remediation by indirect providing of oxygen and stimulation of contaminant biodegradation. This technique is planned for products recovery from remediating capillary, light non-aqueous phase liquids (LNAPLs), unsaturated and saturated zones. This technique used to remediate soils which are contaminated with volatile and semi-volatile organic compounds. The method uses a “slurp” that spreads into the free product layer, which pulls up liquids from this layer.
-

- Biosparging: This technique is similar to bioventing in this air is injected into soil subsurface to improve microbial activities which stimulate pollutant removal from polluted sites. However, in bioventing, air is injected in saturated zone, which can help in upward movement of volatile organic compounds to the unsaturated zone to stimulate biodegradation process. The efficiency of biosparging depends on two major factors specifically soil permeability and pollutant biodegradability.
-
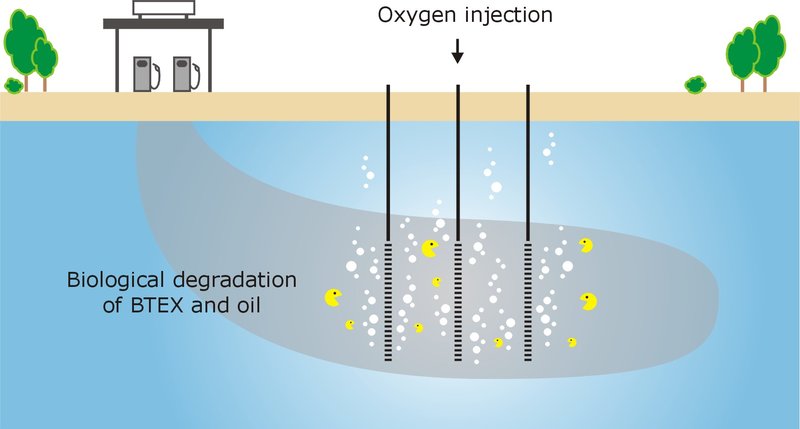
- Biostimulation: Involves the addition of nutrients, electron donors, or electron acceptors to stimulate the existing microbial population to enhance biodegradation rates.
- Bioaugmentation: Involves the addition of specific strains of microorganisms known to degrade contaminants to the site to enhance biodegradation.
-

- Phytoremediation: Phytoremediation is depolluting the contaminated soils. This technique based on plant interactions like physical, chemical, biological, microbiological and biochemical in contaminated sites to diminish the toxic properties of pollutants. Which is depending on pollutant amount and nature, there are several mechanisms such as extraction, degradation, filtration, accumulation, stabilization and volatilization involved in phytoremediation. Pollutants like heavy metals and radionuclides are commonly removed by extraction, transformation and sequestration. Organic pollutants hydrocarbons and chlorinated compounds are mostly removed by degradation, rhizoremediation, stabilization and volatilization, with mineralization being possible when some plants such as willow and alfalfa are used.
-

Advantages:
- Minimal disruption to the site.
- Lower cost compared to ex situ methods.
- Suitable for large and deep contamination zones.
Disadvantages:
- Slower degradation rates.
- Less control over environmental conditions.
- Potentially limited effectiveness for highly toxic or recalcitrant compounds.
2. Ex Situ Biodegradation
Ex situ biodegradation involves the removal of contaminated material to another location for treatment. This method allows for greater control over environmental conditions and treatment processes, potentially leading to faster and more complete degradation of contaminants.
Types of Ex Situ Biodegradation:
- Biopiles: Contaminated soil is excavated and piled, and then aerated, irrigated, and amended with nutrients and microbial inoculants as needed to promote biodegradation. Bioremediation includes above-ground piling of dug polluted soil, followed by aeration and nutrient amendment to improve bioremediation by microbial metabolic activities. This technique comprises aeration, irrigation, nutrients, leachate collection and treatment bed systems.
-
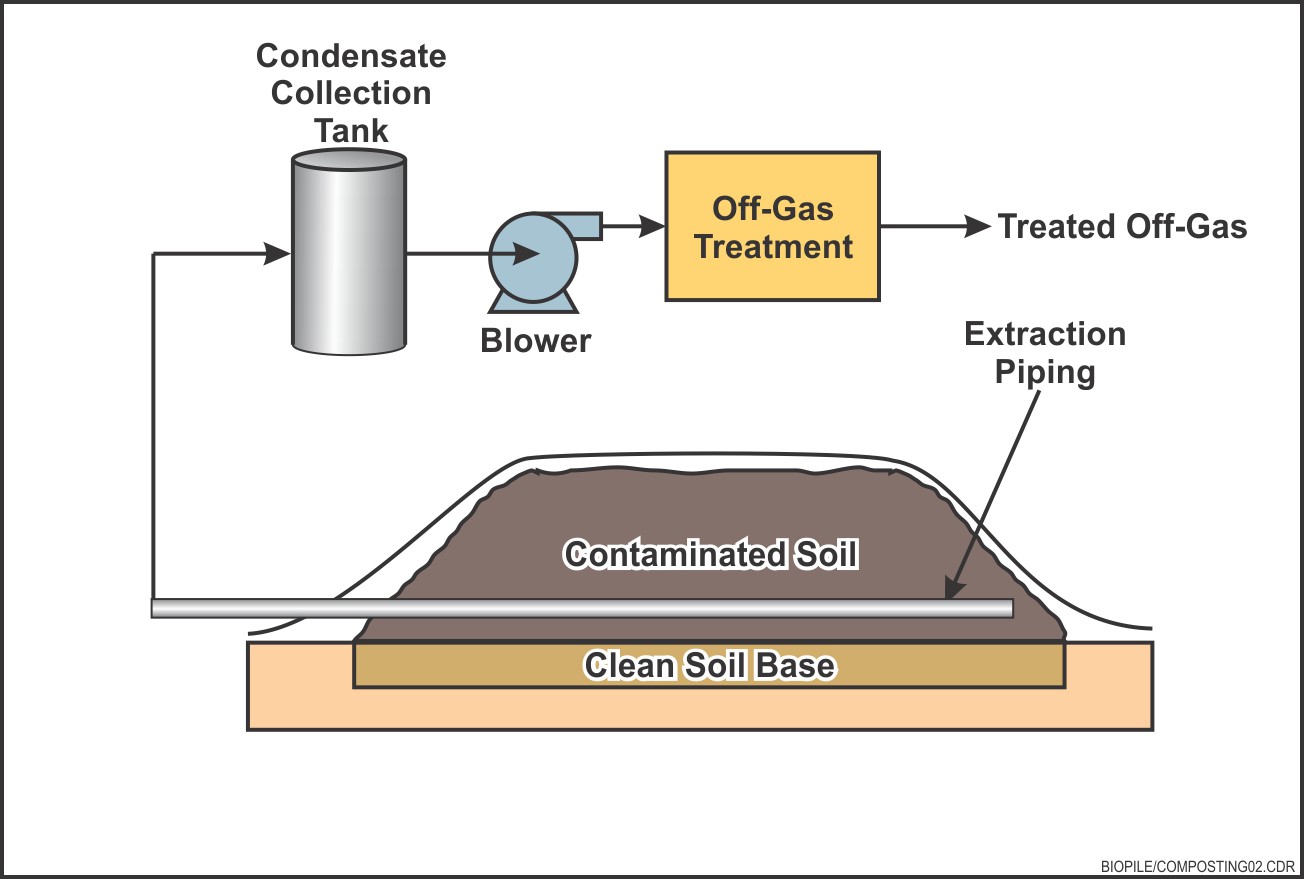
- Windrow Composting: Contaminated soil is formed into long rows (windrows) and regularly turned to aerate the material and promote microbial activity. Windrows is bioremediation techniques depends on periodic rotating the piled polluted soil to improve bioremediation by increasing microbial degradation activities of native and transient hydrocarbonoclastic present in polluted soil. The periodic turning of polluted soil increase in aeration with addition of water, uniform distribution of nutrients, pollutants and microbial degradation activities, accordingly increase the rate of bioremediation, which can be proficient through acclimatization, biotransformation and mineralization.
-

- Landfarming: Contaminated soil is spread over a large area and periodically tilled to aerate the soil and enhance biodegradation. Land farming is the simplest, outstanding bioremediation techniques due to its low cost and less equipment requirement for operation. It is mostly observed in ex-situ bioremediation, while in some cases of in-situ bioremediation technique. This consideration is due to the site of treatment. Pollutant depth is important in land farming which can be carried out ex-situ or in-situ. In land farming, polluted soils are regularly excavated and tilled and site of treatment speciously regulates the type of bioremediation.
-
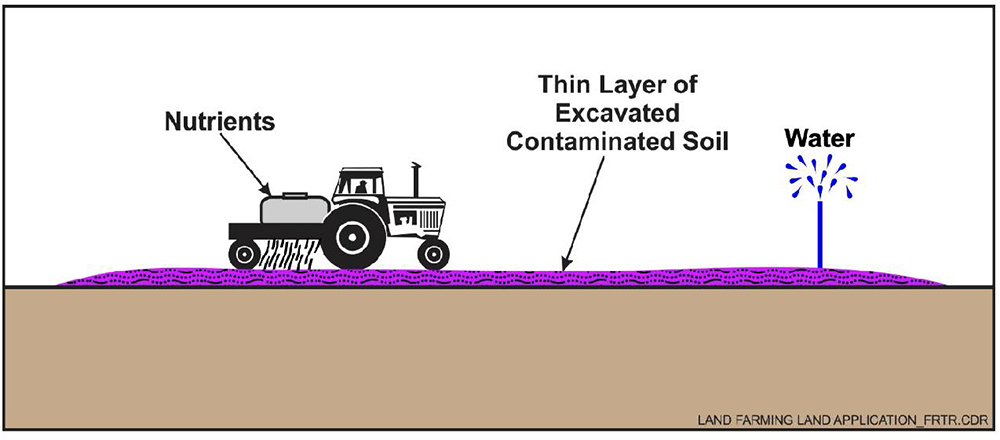
- Bioreactors: Contaminated soil or water is placed in a reactor vessel where environmental conditions (temperature, pH, oxygen levels) are tightly controlled to optimize biodegradation. Bioreactor is a vessel in which raw materials are converted to specific product(s) following series of biological reactions. There are different operational modes of bioreactors, which include: batch, fed-batch, sequencing batch, continuous and multistage. Bioreactor provides optimal growth conditions for bioremediation. Bioreactor filled with polluted samples for remediation process.
-

Advantages:
- Greater control over treatment conditions.
- Faster degradation rates.
- Effective for a wide range of contaminants.
Disadvantages:
- Higher cost due to excavation, transport, and treatment setup.
- Potentially disruptive to the site.
- Requires disposal of treated material.
John Doe
5 min agoLorem ipsum dolor sit amet, consectetur adipisicing elit, sed do eiusmod tempor incididunt ut labore et dolore magna aliqua. Ut enim ad minim veniam, quis nostrud exercitation ullamco laboris nisi ut aliquip ex ea commodo consequat.
ReplyJohn Doe
5 min agoLorem ipsum dolor sit amet, consectetur adipisicing elit, sed do eiusmod tempor incididunt ut labore et dolore magna aliqua. Ut enim ad minim veniam, quis nostrud exercitation ullamco laboris nisi ut aliquip ex ea commodo consequat.
Reply