Factors influencing microbial population

Microbial populations in any given environment are influenced by a wide range of factors, which can be broadly categorized into abiotic (non-living) and biotic (living) factors. Understanding these factors is crucial for managing microbial communities in various contexts, such as agriculture, environmental remediation, and human health.
Abiotic Factors
Temperature:
- Microorganisms have optimal temperature ranges for growth and metabolism.
- Psychrophiles thrive in cold environments, mesophiles in moderate temperatures, and thermophiles in hot conditions.
- Extreme temperatures can denature proteins and nucleic acids, inhibiting microbial growth.
pH:
- Most bacteria prefer neutral pH (6.5-7.5), while fungi can tolerate more acidic conditions.
- Extremes in pH can affect enzyme activity, nutrient availability, and membrane integrity.
- Soil pH influences the solubility of minerals and nutrients, impacting microbial metabolism.
Moisture:
- Water is essential for microbial metabolic processes.
- High moisture levels facilitate microbial activity, but excessive water can lead to anaerobic conditions, favoring anaerobes over aerobes.
- Desiccation can inhibit microbial growth or lead to spore formation in some microorganisms.
Oxygen Availability:
- Aerobic microorganisms require oxygen for respiration, while anaerobes thrive in oxygen-free environments.
- Facultative anaerobes can switch between aerobic and anaerobic metabolism based on oxygen availability.
- Oxygen levels influence redox potential, affecting microbial growth and activity.
Nutrient Availability:
- The presence of carbon, nitrogen, phosphorus, and other essential nutrients determines microbial growth and population size.
- Nutrient-rich environments support higher microbial biomass and diversity.
- Limiting nutrients can constrain microbial growth and lead to competition among microorganisms.
Salinity:
- Microorganisms have varying tolerances to salt concentrations.
- Halophiles thrive in high-salt environments, whereas most microorganisms prefer lower salinity levels.
- High salinity can lead to osmotic stress, affecting microbial cell function.
Light:
- Photosynthetic microorganisms, such as cyanobacteria and algae, require light for energy production.
- Light availability influences the composition and activity of phototrophic microbial communities.
- UV light can have detrimental effects, causing DNA damage in exposed microorganisms.
Biotic Factors
Interactions with Other Microorganisms:
- Competition: Microorganisms compete for nutrients, space, and resources. Competitive exclusion can limit the diversity of microbial populations.
- Symbiosis: Mutualistic relationships, such as those between mycorrhizal fungi and plant roots, enhance nutrient uptake and microbial growth.
- Predation: Protozoa, nematodes, and other organisms can prey on microorganisms, regulating their populations.
- Parasitism: Pathogenic microorganisms can infect and reduce populations of other microbes.
Plant-Microbe Interactions:
- Plants release root exudates that provide nutrients and signaling molecules, promoting the growth of specific microbial populations.
- Rhizosphere interactions influence nutrient cycling, plant health, and soil structure.
- Mycorrhizal associations enhance nutrient uptake for plants and provide carbohydrates for fungi.
Animal-Microbe Interactions:
- Animals contribute organic matter through waste products, influencing microbial activity and nutrient cycling.
- Gut microbiomes in animals play crucial roles in digestion, immunity, and overall health.
- Animal grazing can impact microbial communities by altering plant cover and soil structure.
Environmental and Anthropogenic Factors
Soil Type and Structure:
- Soil texture, porosity, and aggregate stability affect water retention, aeration, and nutrient availability, influencing microbial habitats.
- Organic matter content and soil amendments can enhance microbial activity and diversity.
Land Use and Agricultural Practices:
- Crop rotation, tillage, fertilization, and pesticide application impact microbial populations and soil health.
- Sustainable practices, such as organic farming and conservation tillage, promote beneficial microbial communities.
Pollution and Contamination:
- Chemical pollutants, heavy metals, and organic contaminants can inhibit microbial growth or select for resistant populations.
- Bioremediation efforts utilize specific microorganisms to degrade pollutants and restore contaminated environments.
Climate Change:
- Changes in temperature, precipitation, and CO2 levels affect microbial metabolism, distribution, and community dynamics.
- Extreme weather events can disrupt microbial habitats and nutrient cycling processes.
Monitoring and Managing Microbial Populations
Molecular Techniques:
- DNA sequencing, metagenomics, and transcriptomics provide insights into microbial diversity, functions, and interactions.
- Quantitative PCR (qPCR) and fluorescence in situ hybridization (FISH) are used to quantify specific microbial populations.
Cultivation-Based Methods:
- Isolation and culturing of microorganisms on selective media help identify and study microbial physiology and interactions.
- Enrichment cultures enhance the growth of specific microorganisms for further analysis.
Biostimulation and Bioaugmentation:
- Biostimulation involves adding nutrients or amendments to stimulate indigenous microbial activity.
- Bioaugmentation introduces specific microbial strains to enhance bioremediation or soil health.
Sustainable Practices:
- Implementing sustainable agricultural and land use practices supports healthy microbial communities and ecosystem functions.
- Reducing chemical inputs and promoting biodiversity enhance microbial resilience and activity.
Distribution of different types of soil microorganisms

Soil microorganisms are critical for nutrient cycling, soil fertility, and plant health. Their distribution in soil is influenced by various environmental factors, soil properties, and plant interactions. Understanding the distribution of these microorganisms helps in managing soil health and improving agricultural productivity.
Types of Soil Microorganisms
Bacteria:
- General Characteristics:
- Single-celled, prokaryotic organisms.
- Most abundant microorganisms in soil, with populations ranging from millions to billions per gram of soil.
- Functional Groups:
- Decomposers: Break down organic matter (e.g., Bacillus, Pseudomonas).
- Nitrogen-fixing Bacteria: Convert atmospheric nitrogen to ammonia (e.g., Rhizobium, Azotobacter).
- Nitrifying Bacteria: Convert ammonia to nitrate (e.g., Nitrosomonas, Nitrobacter).
- Denitrifying Bacteria: Convert nitrate to nitrogen gas (e.g., Pseudomonas, Clostridium).
- Phosphate-solubilizing Bacteria: Release inorganic phosphate from organic compounds (e.g., Bacillus, Pseudomonas).
Fungi:
- General Characteristics:
- Eukaryotic organisms, including yeasts, molds, and mushrooms.
- Play a crucial role in decomposing complex organic compounds like cellulose and lignin.
- Functional Groups:
- Saprophytic Fungi: Decompose dead organic matter (e.g., Aspergillus, Penicillium).
- Mycorrhizal Fungi: Form symbiotic relationships with plant roots, enhancing nutrient uptake (e.g., Glomus, Rhizophagus).
- Pathogenic Fungi: Cause diseases in plants (e.g., Fusarium, Phytophthora).
Actinomycetes:
- General Characteristics:
- Filamentous bacteria that resemble fungi.
- Produce antibiotics and play a role in decomposing complex organic matter.
- Functional Groups:
- Decomposers: Break down recalcitrant organic compounds like chitin and lignocellulose (e.g., Streptomyces).
Algae:
- General Characteristics:
- Photosynthetic, eukaryotic organisms found in soil moisture.
- Contribute to soil fertility by fixing atmospheric carbon through photosynthesis.
- Functional Groups:
- Green Algae (Chlorophyta): Common in moist soils.
- Cyanobacteria (Blue-Green Algae): Capable of nitrogen fixation (e.g., Anabaena, Nostoc).
Protozoa:
- General Characteristics:
- Single-celled, eukaryotic organisms.
- Feed on bacteria and other microorganisms, regulating microbial populations.
- Functional Groups:
- Amoebae: Move and feed using pseudopodia.
- Flagellates: Use whip-like flagella for movement.
- Ciliates: Use hair-like cilia for locomotion and feeding.
Nematodes:
- General Characteristics:
- Microscopic, worm-like organisms.
- Play roles in nutrient cycling, plant health, and disease suppression.
- Functional Groups:
- Bacterivores: Feed on bacteria.
- Fungivores: Feed on fungi.
- Predators: Feed on other nematodes and microorganisms.
- Plant Parasites: Cause damage to plant roots.
Factors Influencing Microbial Distribution in Soil
Soil Type and Texture:
- Sandy soils tend to have lower microbial biomass due to poor water and nutrient retention.
- Clayey soils support higher microbial populations due to better water and nutrient holding capacity.
- Loamy soils, with a balance of sand, silt, and clay, are generally the most fertile and support diverse microbial communities.
Soil pH:
- Soil pH affects the availability of nutrients and the composition of microbial communities.
- Most bacteria thrive in neutral to slightly alkaline soils (pH 6.5-7.5).
- Fungi are more tolerant of acidic conditions and can dominate in low pH soils.
Organic Matter:
- Organic matter provides a food source for microorganisms.
- High levels of organic matter support larger and more diverse microbial populations.
- Compost and organic amendments enhance microbial activity and diversity.
Moisture:
- Adequate soil moisture is essential for microbial metabolism and activity.
- Waterlogged soils may favor anaerobic microorganisms like denitrifiers and sulfate reducers.
- Dry soils may reduce microbial activity and biomass.
Temperature:
- Soil temperature influences microbial growth rates and activity.
- Most soil microorganisms are mesophilic, thriving at moderate temperatures (20-30°C).
- Extreme temperatures can inhibit microbial activity or select for thermophilic or psychrophilic species.
Nutrient Availability:
- The presence of essential nutrients like carbon, nitrogen, phosphorus, and sulfur supports microbial growth.
- Fertilization and soil amendments can influence microbial populations and activity.
Plant Root Exudates:
- Plants release a variety of organic compounds through their roots, known as root exudates.
- These exudates attract and stimulate specific microbial populations in the rhizosphere.
- Different plant species and genotypes can shape distinct rhizosphere microbial communities.
Soil Aeration:
- Aerobic microorganisms require oxygen for respiration and are dominant in well-aerated soils.
- Anaerobic microorganisms thrive in oxygen-depleted conditions, such as waterlogged soils.
- Soil structure and porosity influence oxygen diffusion and microbial distribution.
Distribution Patterns of Soil Microorganisms
Horizontal Distribution:
- Microbial populations can vary across different land uses, such as agricultural fields, forests, grasslands, and urban areas.
- Agricultural soils may have higher bacterial populations due to regular inputs of organic matter and fertilizers.
- Forest soils often have abundant fungi due to the decomposition of leaf litter and woody debris.
Vertical Distribution:
- Microbial populations decrease with soil depth due to reduced organic matter and root exudates.
- Surface soils (topsoil) have the highest microbial activity and diversity.
- Subsurface soils (subsoil) may harbor specialized microbial communities adapted to lower nutrient availability and different environmental conditions.
Temporal Distribution:
- Microbial populations and activity can vary seasonally with changes in temperature, moisture, and plant growth cycles.
- Seasonal changes in plant cover and root exudation patterns influence microbial dynamics in the rhizosphere.
Factors influencing microbial population

Microbial populations in any given environment are influenced by a wide range of factors, which can be broadly categorized into abiotic (non-living) and biotic (living) factors. Understanding these factors is crucial for managing microbial communities in various contexts, such as agriculture, environmental remediation, and human health.
Abiotic Factors
Temperature:
- Microorganisms have optimal temperature ranges for growth and metabolism.
- Psychrophiles thrive in cold environments, mesophiles in moderate temperatures, and thermophiles in hot conditions.
- Extreme temperatures can denature proteins and nucleic acids, inhibiting microbial growth.
pH:
- Most bacteria prefer neutral pH (6.5-7.5), while fungi can tolerate more acidic conditions.
- Extremes in pH can affect enzyme activity, nutrient availability, and membrane integrity.
- Soil pH influences the solubility of minerals and nutrients, impacting microbial metabolism.
Moisture:
- Water is essential for microbial metabolic processes.
- High moisture levels facilitate microbial activity, but excessive water can lead to anaerobic conditions, favoring anaerobes over aerobes.
- Desiccation can inhibit microbial growth or lead to spore formation in some microorganisms.
Oxygen Availability:
- Aerobic microorganisms require oxygen for respiration, while anaerobes thrive in oxygen-free environments.
- Facultative anaerobes can switch between aerobic and anaerobic metabolism based on oxygen availability.
- Oxygen levels influence redox potential, affecting microbial growth and activity.
Nutrient Availability:
- The presence of carbon, nitrogen, phosphorus, and other essential nutrients determines microbial growth and population size.
- Nutrient-rich environments support higher microbial biomass and diversity.
- Limiting nutrients can constrain microbial growth and lead to competition among microorganisms.
Salinity:
- Microorganisms have varying tolerances to salt concentrations.
- Halophiles thrive in high-salt environments, whereas most microorganisms prefer lower salinity levels.
- High salinity can lead to osmotic stress, affecting microbial cell function.
Light:
- Photosynthetic microorganisms, such as cyanobacteria and algae, require light for energy production.
- Light availability influences the composition and activity of phototrophic microbial communities.
- UV light can have detrimental effects, causing DNA damage in exposed microorganisms.
Biotic Factors
Interactions with Other Microorganisms:
- Competition: Microorganisms compete for nutrients, space, and resources. Competitive exclusion can limit the diversity of microbial populations.
- Symbiosis: Mutualistic relationships, such as those between mycorrhizal fungi and plant roots, enhance nutrient uptake and microbial growth.
- Predation: Protozoa, nematodes, and other organisms can prey on microorganisms, regulating their populations.
- Parasitism: Pathogenic microorganisms can infect and reduce populations of other microbes.
Plant-Microbe Interactions:
- Plants release root exudates that provide nutrients and signaling molecules, promoting the growth of specific microbial populations.
- Rhizosphere interactions influence nutrient cycling, plant health, and soil structure.
- Mycorrhizal associations enhance nutrient uptake for plants and provide carbohydrates for fungi.
Animal-Microbe Interactions:
- Animals contribute organic matter through waste products, influencing microbial activity and nutrient cycling.
- Gut microbiomes in animals play crucial roles in digestion, immunity, and overall health.
- Animal grazing can impact microbial communities by altering plant cover and soil structure.
Environmental and Anthropogenic Factors
Soil Type and Structure:
- Soil texture, porosity, and aggregate stability affect water retention, aeration, and nutrient availability, influencing microbial habitats.
- Organic matter content and soil amendments can enhance microbial activity and diversity.
Land Use and Agricultural Practices:
- Crop rotation, tillage, fertilization, and pesticide application impact microbial populations and soil health.
- Sustainable practices, such as organic farming and conservation tillage, promote beneficial microbial communities.
Pollution and Contamination:
- Chemical pollutants, heavy metals, and organic contaminants can inhibit microbial growth or select for resistant populations.
- Bioremediation efforts utilize specific microorganisms to degrade pollutants and restore contaminated environments.
Climate Change:
- Changes in temperature, precipitation, and CO2 levels affect microbial metabolism, distribution, and community dynamics.
- Extreme weather events can disrupt microbial habitats and nutrient cycling processes.
Monitoring and Managing Microbial Populations
Molecular Techniques:
- DNA sequencing, metagenomics, and transcriptomics provide insights into microbial diversity, functions, and interactions.
- Quantitative PCR (qPCR) and fluorescence in situ hybridization (FISH) are used to quantify specific microbial populations.
Cultivation-Based Methods:
- Isolation and culturing of microorganisms on selective media help identify and study microbial physiology and interactions.
- Enrichment cultures enhance the growth of specific microorganisms for further analysis.
Biostimulation and Bioaugmentation:
- Biostimulation involves adding nutrients or amendments to stimulate indigenous microbial activity.
- Bioaugmentation introduces specific microbial strains to enhance bioremediation or soil health.
Sustainable Practices:
- Implementing sustainable agricultural and land use practices supports healthy microbial communities and ecosystem functions.
- Reducing chemical inputs and promoting biodiversity enhance microbial resilience and activity.
Rhizosphere effect
The rhizosphere is the narrow region of soil directly influenced by root secretions and associated soil microorganisms. This zone is characterized by a high microbial density and activity, influenced by the plant roots' physical, chemical, and biological interactions with the soil environment. The rhizosphere effect refers to the enhanced microbial activity and diversity in this region compared to bulk soil (soil not influenced by roots).

Key Aspects of the Rhizosphere Effect 
Root Exudates:
- Plant roots release a variety of compounds, known as root exudates, including sugars, amino acids, organic acids, vitamins, and enzymes.
- These exudates serve as energy sources and nutrients for soil microorganisms, promoting their growth and activity.
Microbial Populations:
- The rhizosphere harbors diverse microbial communities, including bacteria, fungi, archaea, protozoa, and nematodes.
- Beneficial microorganisms, such as nitrogen-fixing bacteria, phosphate-solubilizing bacteria, and mycorrhizal fungi, are often more abundant in the rhizosphere.
Microbial Interactions:
- The rhizosphere is a hotspot for various microbial interactions, including competition, cooperation, predation, and symbiosis.
- These interactions can influence nutrient cycling, plant health, and soil structure.
Nutrient Cycling:
- Microbial activity in the rhizosphere enhances the cycling of essential nutrients, such as nitrogen, phosphorus, and sulfur, making them more available to plants.
- Decomposition of organic matter by rhizosphere microorganisms releases nutrients that plants can absorb.
Factors Influencing the Rhizosphere Effect
Plant Species and Genotype:
- Different plant species and even different genotypes within a species can produce varying quantities and types of root exudates, affecting the composition and activity of rhizosphere microbial communities.
Soil Type and Properties:
- Soil pH, texture, organic matter content, and nutrient availability influence the microbial populations and their activity in the rhizosphere.
Root Architecture:
- Root length, density, and surface area affect the extent of the rhizosphere and the distribution of exudates, influencing microbial colonization and activity.
Environmental Conditions:
- Temperature, moisture, and oxygen levels impact microbial growth and activity in the rhizosphere.
Benefits of the Rhizosphere Effect
Enhanced Nutrient Uptake:
- Microbial processes in the rhizosphere improve the availability and uptake of essential nutrients by plants, promoting better growth and development.
Disease Suppression:
- Certain rhizosphere microorganisms produce antibiotics, antifungal compounds, and other bioactive molecules that suppress soil-borne pathogens and protect plants from diseases.
Plant Growth Promotion:
- Beneficial microbes, such as plant growth-promoting rhizobacteria (PGPR), enhance plant growth through various mechanisms, including hormone production, nitrogen fixation, and stress alleviation.
Soil Health and Structure:
- Microbial activity in the rhizosphere contributes to soil aggregation, organic matter decomposition, and overall soil health, improving soil structure and fertility.
Applications and Future Prospects
Agriculture:
- Leveraging the rhizosphere effect through the use of biofertilizers, biopesticides, and soil amendments can enhance crop productivity and sustainability.
- Developing crop varieties that can efficiently interact with beneficial rhizosphere microorganisms is a promising area of research.
Environmental Remediation:
- Rhizosphere microorganisms can be utilized in bioremediation efforts to degrade environmental pollutants and restore contaminated soils.
Soil Health Management:
- Understanding and managing the rhizosphere effect is crucial for maintaining soil health, enhancing nutrient cycling, and promoting sustainable land use practices.
Challenges and Research Directions
Complexity of Microbial Communities:
- The rhizosphere is a complex and dynamic environment with highly diverse microbial communities, making it challenging to unravel specific interactions and functions.
Plant-Microbe Interactions:
- Further research is needed to understand the molecular mechanisms underlying plant-microbe interactions in the rhizosphere and their impact on plant health and soil fertility.
Environmental Variability:
- The rhizosphere effect can vary widely depending on environmental conditions, necessitating research on how climate change and land use practices influence rhizosphere dynamics.
Technological Advancements:
- Advances in genomics, metagenomics, transcriptomics, and other omics technologies are providing new insights into the rhizosphere microbiome and its functions, opening up new avenues for research and application.
Microbes in decomposition, mineralisation and recycling process
Microorganisms play a crucial role in the decomposition of organic matter, mineralization of nutrients, and the recycling of elements within ecosystems. Their activities ensure the continuous availability of essential nutrients for plant and animal life and help maintain ecosystem stability.
Decomposition
Decomposition is the process by which organic substances are broken down into simpler organic or inorganic matter. It is a key step in the recycling of nutrients in ecosystems.
Key Microorganisms Involved in Decomposition
Bacteria:
- Bacteria are primary decomposers of dead plant and animal tissues.
- They secrete extracellular enzymes that break down complex organic compounds such as cellulose, hemicellulose, proteins, and lipids into simpler compounds.
- Examples include Bacillus, Pseudomonas, and Streptomyces species.
Fungi:
- Fungi are efficient decomposers, particularly of complex plant materials like lignin and cellulose.
- Saprophytic fungi, such as Aspergillus, Penicillium, and Trichoderma, play a significant role in decomposing organic matter in soil and leaf litter.
- White-rot fungi and brown-rot fungi are specialized in breaking down lignin and cellulose, respectively.
Actinomycetes:
- Actinomycetes, such as Streptomyces species, decompose complex organic compounds, producing a characteristic earthy smell in soil.
- They are particularly important in degrading tough organic materials like chitin and lignocellulose.
Protozoa and Nematodes:
- These microorganisms consume bacteria and fungi, regulating microbial populations and recycling nutrients back into the soil.
Process of Decomposition
Leaching:
- Water-soluble substances are leached out from decomposing organic matter, making them available for microbial uptake.
Fragmentation:
- Soil fauna such as earthworms, insects, and other detritivores break down organic matter into smaller pieces, increasing the surface area for microbial action.
Catabolism:
- Microorganisms secrete enzymes to break down complex organic compounds into simpler molecules.
- This involves both aerobic and anaerobic metabolic pathways, depending on the availability of oxygen.
Humification:
- The formation of humus, a stable organic matter, results from the microbial and chemical transformation of organic residues.
- Humus enhances soil structure, water retention, and nutrient-holding capacity.
Mineralization
Mineralization is the conversion of organic compounds into inorganic forms, making nutrients available for plant uptake.
Key Processes in Mineralization
Nitrogen Mineralization:
- Proteins and nucleic acids in organic matter are broken down by bacteria and fungi into ammonium (NH4+).
- Ammonium can be further oxidized by nitrifying bacteria into nitrate (NO3-), a process known as nitrification.
- Examples of microorganisms involved include Nitrosomonas and Nitrobacter species.
Phosphorus Mineralization:
- Organic phosphorus compounds are decomposed by phosphate-solubilizing bacteria and fungi into inorganic phosphate.
- Enzymes such as phosphatases play a crucial role in this process.
- Examples include Pseudomonas, Bacillus, and Aspergillus species.
Sulfur Mineralization:
- Organic sulfur compounds are converted into sulfate (SO4^2-) by sulfur-oxidizing bacteria.
- Examples include Thiobacillus and Desulfovibrio species.
Recycling
Recycling involves the return of nutrients to the ecosystem, ensuring their continuous availability.
Key Microbial Processes in Recycling
Carbon Cycle:
- Decomposers break down dead organic matter, releasing carbon dioxide (CO2) through respiration.
- Some carbon is incorporated into microbial biomass and soil organic matter, contributing to soil carbon storage.
Nitrogen Cycle:
- Nitrogen-fixing bacteria (e.g., Rhizobium, Azotobacter) convert atmospheric nitrogen (N2) into ammonia (NH3), making it available to plants.
- Decomposers mineralize organic nitrogen, while nitrifying bacteria convert ammonia to nitrate.
- Denitrifying bacteria (e.g., Pseudomonas, Clostridium) convert nitrate back to N2, completing the nitrogen cycle.
Phosphorus Cycle:
- Phosphate-solubilizing microorganisms release inorganic phosphate from organic compounds.
- Mycorrhizal fungi enhance phosphate uptake by plant roots, forming symbiotic associations.
Sulfur Cycle:
- Sulfur-oxidizing bacteria convert hydrogen sulfide (H2S) into sulfate, which can be assimilated by plants.
- Sulfate-reducing bacteria convert sulfate back to sulfide in anaerobic conditions.
Bioremediation

Bioremediation is a process that uses microorganisms, plants, or microbial enzymes to detoxify, degrade, and remove pollutants from the environment. It is an environmentally friendly and cost-effective method for cleaning up contaminated sites, such as soil, water, and air.
Principles of Bioremediation
Microbial Metabolism:
- Microorganisms (bacteria, fungi, algae) metabolize pollutants, using them as energy sources or converting them into harmless by-products.
- The metabolic processes can be aerobic (requiring oxygen) or anaerobic (occurring in the absence of oxygen).
Natural Attenuation:
- This process relies on natural biodegradation activities in the environment without human intervention. Over time, microorganisms naturally degrade contaminants.
Bioaugmentation:
- The introduction of specific strains of microorganisms that are capable of degrading the contaminants to enhance the bioremediation process.
Biostimulation:
- The addition of nutrients, oxygen, or other substances to stimulate the growth and activity of indigenous microorganisms to enhance the bioremediation process.
Types of Bioremediation
1. In Situ Bioremediation:
In situ bioremediation occurs directly at the contaminated site, avoiding the need to excavate or transport contaminated material.
- Bioventing: Enhancing the activity of indigenous microorganisms by supplying air or oxygen to the subsurface.
- Biosparging: Injection of air or oxygen into the saturated zone to stimulate aerobic degradation of contaminants in groundwater.
- Phytoremediation: Use of plants to absorb, accumulate, and degrade contaminants from soil and water.
- Natural Attenuation: Reliance on natural processes and indigenous microorganisms to degrade contaminants over time.
2. Ex Situ Bioremediation:
Ex situ bioremediation involves the removal of contaminated material to another location for treatment.
- Biopiles: Contaminated soil is excavated, piled, and aerated to enhance microbial activity.
- Landfarming: Contaminated soil is spread over a large area and periodically tilled to aerate and stimulate microbial degradation.
- Composting: Mixing contaminated soil with organic materials to enhance microbial activity and biodegradation.
- Bioreactors: Contaminated material is placed in a reactor vessel where environmental conditions are controlled to optimize biodegradation.
Applications of Bioremediation
Oil Spill Cleanup:
- Bioremediation is used to degrade hydrocarbons in oil spills, reducing environmental damage to marine and terrestrial ecosystems.
Industrial Waste Treatment:
- Effective for treating industrial effluents and waste containing organic pollutants, heavy metals, and other toxic substances.
Agricultural Waste Management:
- Degrades pesticides, herbicides, and fertilizers in agricultural runoff, reducing their impact on soil and water quality.
Groundwater Remediation:
- Removes contaminants such as solvents, petroleum hydrocarbons, and heavy metals from groundwater.
Wastewater Treatment:
- Biological treatment processes in wastewater treatment plants use microorganisms to degrade organic matter and pollutants.
Advantages of Bioremediation
- Environmentally Friendly: Utilizes natural processes and minimizes the use of harmful chemicals.
- Cost-Effective: Often more affordable than physical or chemical remediation methods.
- Versatile: Applicable to a wide range of contaminants and environmental settings.
- Sustainable: Promotes the natural restoration of ecosystems.
Limitations of Bioremediation
- Time-Consuming: Can take longer to achieve desired cleanup levels compared to other methods.
- Specific Conditions Required: Effectiveness depends on suitable environmental conditions (e.g., pH, temperature, oxygen levels).
- Incomplete Degradation: Some contaminants may not be fully degraded or may produce harmful by-products.
- Limited Scope: Not effective for all types of contaminants, particularly non-biodegradable substances.
Solubilisation of phosphates
Phosphate solubilization is the process by which insoluble forms of phosphate are converted into soluble forms that can be readily absorbed by plants and microorganisms. This process is crucial for plant nutrition and soil fertility, as phosphorus is a key nutrient that often limits plant growth. In microbiology, certain microorganisms, known as phosphate-solubilizing microorganisms (PSMs), play a vital role in this process.
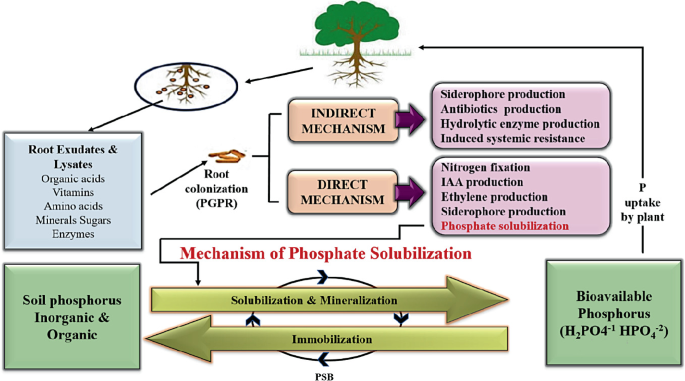
Importance of Phosphate Solubilization
Phosphorus is a major essential nutrient for plants, involved in various physiological processes including energy transfer, photosynthesis, and nutrient movement within the plant. Despite its abundance in the soil, much of it is in insoluble forms that plants cannot uptake. Phosphate solubilization by microorganisms helps in converting these insoluble forms into bioavailable forms, enhancing plant growth and agricultural productivity.
Mechanisms of Phosphate Solubilization
Phosphate solubilizing microorganisms employ various mechanisms to solubilize phosphates:
Production of Organic Acids:
- Microorganisms produce organic acids such as citric acid, oxalic acid, lactic acid, acetic acid, gluconic acid, and malic acid.
- These acids lower the pH of the surrounding environment, which leads to the dissolution of insoluble phosphate compounds, releasing phosphate ions into the soil solution.
Enzymatic Activity:
- Enzymes such as phosphatases, phytases, and C-P lyases are produced by microorganisms.
- These enzymes hydrolyze organic phosphorus compounds into inorganic phosphate, making it available for plant uptake.
Chelation:
- Microorganisms produce chelating agents (siderophores) that bind to metal ions such as calcium, iron, and aluminum, which are often associated with phosphate, forming soluble complexes and releasing phosphate ions.
Ion Exchange:
- Certain microbial metabolites can exchange ions with insoluble phosphate compounds, leading to the release of phosphate ions.
Redox Reactions:
- Some microorganisms can alter the oxidation state of phosphorus, facilitating its solubilization.
Types of Phosphate-Solubilizing Microorganisms
Bacteria:
- Pseudomonas spp., Bacillus spp., Rhizobium spp., Enterobacter spp., and Azospirillum spp. are well-known phosphate-solubilizing bacteria.
- These bacteria are often found in the rhizosphere, the soil region near plant roots, where they interact with plant roots and enhance phosphorus availability.
Fungi:
- Aspergillus spp., Penicillium spp., and Trichoderma spp. are notable phosphate-solubilizing fungi.
- Fungi can solubilize phosphate more effectively than bacteria due to their extensive hyphal networks, which can explore a larger soil volume.
Actinomycetes:
- Certain actinomycetes like Streptomyces spp. also possess phosphate-solubilizing capabilities.
Applications of Phosphate-Solubilizing Microorganisms
Agriculture:
- PSMs are used as biofertilizers to enhance phosphorus availability in the soil, promoting plant growth and increasing crop yields.
- They can be applied to seeds, soil, or as part of composts and manures.
Sustainable Farming:
- Use of PSMs reduces the dependency on chemical phosphate fertilizers, leading to sustainable and eco-friendly farming practices.
- They help in maintaining soil health and fertility over the long term.
Rehabilitation of Degraded Lands:
- PSMs can be used in the rehabilitation of degraded and phosphorus-deficient soils, improving soil structure and fertility.
Challenges and Future Prospects
Variability in Field Conditions:
- The effectiveness of PSMs can vary widely depending on soil type, pH, temperature, moisture, and the presence of other microorganisms.
- Understanding the interactions between PSMs and the soil environment is crucial for optimizing their application.
Strain Selection and Genetic Improvement:
- Selecting efficient strains and genetically enhancing PSMs for higher phosphate solubilization potential is an ongoing area of research.
- Genetic engineering and synthetic biology approaches are being explored to develop more effective PSM strains.
Formulation and Delivery:
- Developing stable and effective formulations for the delivery of PSMs to the field is a significant challenge.
- Research is focused on improving the shelf life, viability, and efficacy of PSM formulations.
John Doe
5 min agoLorem ipsum dolor sit amet, consectetur adipisicing elit, sed do eiusmod tempor incididunt ut labore et dolore magna aliqua. Ut enim ad minim veniam, quis nostrud exercitation ullamco laboris nisi ut aliquip ex ea commodo consequat.
ReplyJohn Doe
5 min agoLorem ipsum dolor sit amet, consectetur adipisicing elit, sed do eiusmod tempor incididunt ut labore et dolore magna aliqua. Ut enim ad minim veniam, quis nostrud exercitation ullamco laboris nisi ut aliquip ex ea commodo consequat.
Reply